—The periodic table, a product of the collective creativity of science classrooms in the nineteenth century.—
The periodic system is one of the most popular icons of contemporary chemistry. It is a classification of the elements that, through various graphic representations, is used as an educational tool in teaching of chemistry. It is not a simple ordering, since the periodic system also has a predictive capacity. From the second half of the nineteenth century to the present there have been innumerable discoveries of new elements that have confirmed these predictions. From the second half of the nineteenth century, there have been countless discoveries that have confirmed these predictions. However, it is also true that there have been many more unsuccessful than successful predictions, from elements that were supposed to be part of the Sun’s corona, and were never found, to substances with extraordinary properties supposed to revolutionise the chemical industry. Only the predictions, like those of gallium, germanium or scandium, which were finally confirmed in one form or another are remembered. Since its creation in the second half of the nineteenth century, a large number of interpretations have been formulated about the periodic table to explain the causes of the regularities and possibilities of predicting new elements. In the twentieth century, these interpretations made it possible to foster discussions on the reduction of chemistry to the principles of physics, in particular quantum mechanics, the most common interpretative tool of the periodic table from the works of Niels Bohr (1885-1962).
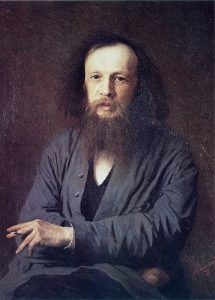
Dimitri Mendeleev in a portrait of 1878. Wikipedia.
Given its role in the teaching of chemistry, it is common to find accounts of its discovery in the manuals of this science. The number of protagonists in the narrative varies, but one of them usually occupies a central position: Dmitri Ivanovich Mendeleev (1834-1907). In one of the most widespread versions, the periodic order was the product of one of Mendeliev’s dreams, during which the new order of the elements was suddenly revealed to him. Another popular narrative states that the Russian chemist elaborated this classification through a card game, a kind of ‘chemical solitaire’, in which Mendeleev wrote down the names of the elements with their periodic properties. The first versions of Mendeleev’s periodic table were presented in March 1869 at one of the sessions of the Russian Chemical Society, which has led to 2019 being declared as the International Year of the Periodic Table of Chemical Elements, endorsed UNESCO.
Actually, it is not possible to place such a complex discovery as the periodic system at a particular point in space and time. Mendeleev was neither the first nor the only person to suggest such classifications. There was a wide range of similar proposals. Among the best known today are those made by Alexandre E. Beguyer de Chancourtois (1819-1866), John Newlands (1837-1898), William Odling (1829-1921), Gustavus Hinrichs (1836-1923), Julius Lothar Meyer (1830-1895). There were many other anonymous professors (Mendeliev was part of this group in 1869) who made proposals in this direction. Actually, more than a multiple discovery, the periodic table was the result of a type of collective creativity typical of science classrooms.
Classifications of chemical substances are rooted in the times of alchemy. During the eighteenth century, affinity tables represented a way of dealing with these problems with enormous teaching potential. The role they played in classrooms was similar to the one the periodic table would play a century later, and at the same time, that they also served to give coherence and sequence the content. However, the classification criteria, as well as the materials classified and the objectives pursued, were very different. Affinity tables aimed to show the interaction between chemicals in order to predict the development of reactions, not the discovery of new substances. They were introduced at the beginning of the century by Etienne-François Geoffroy and, after several decades of relative stability, the tables were expanded in the 1760s and 1770s, so that, like the periodic table, they were constantly changing entities in order to incorporate new data and chemicals. They became so popular that the writer Goethe wrote an account of intertwined love under the title of Elective Affinities (Die Wahlverwandtschaften) in the early nineteenth century. The story was published, paradoxically, when the affinity tables were no longer part of the visual culture of the chemistry classrooms. They progressively disappeared from the collective memory of the academic community of the nineteenth century, leaving no trace in the science manuals of the following century.
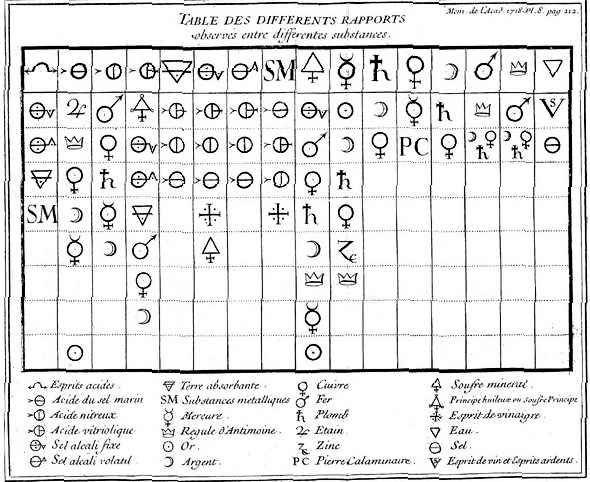
Table of affinities or of the different rapports observed between different substances by Etienne-François Geoffroy (1718). Wikipedia.
When comparing the affinity tables to the periodic tables it can be seen that scientific classifications can have very different foundations. Moreover, even within the same classification criterion, there are always sequencing alternatives, depending on the objectives pursued. One can long for the discovery of the natural order of things, to establish a natural classification based on various conveniently chosen characters. Or, more modestly, one can choose a crucial feature (atomic mass, valence, reactivity with oxygen, etc.) to establish an artificial order. Another even more complex issue is the relationship between natural and artificial classifications and the most appropriate sequencing for pedagogical objectives. Most of the teachers of the mid-nineteenth century preferred to choose hybrid classifications, halfway between the natural and artificial ones because they were more suitable for the teaching of chemistry. Among other issues, they had to address the exponential increase in the number of chemicals, from several hundred at the end of the eighteenth century to several tens of thousands in the nineteenth century, to continue growing in the twentieth century to reach millions. In the twenty-first century, the figure is close to two hundred million, which poses enormous challenges for the teaching of chemistry and the regulation of these substances.
The challenges of the exponential growth of chemicals were very palpable in the mid-nineteenth century, with the arrival of new methods of mineral analysis (such as the spectroscope) and the development of organic synthesis. In this way, the teachers of those years inevitably had to address the problem of sequencing to prepare their lessons or write their manuals. In the middle of the century, especially in the 1860s, several authors combined data on atomic weights with natural classifications to suggest orders that would be the basis of the current periodic system. This situation was partly possible thanks to the partial agreements on atomic weights reached at the Karlsruhe Congress, one of the most important science meetings of those years. This thus explains how the aforementioned authors, including Mendeleev, made proposals for periodic tables based on the increasing order of atomic weights during the 1860s. In those years, Mendeleev was part of the community of ‘invisible teachers’ who taught chemistry throughout Europe. He had just started his career in St. Petersburg, where he started to write a textbook on inorganic chemistry, almost at the same time when he was working his first version of the periodic table. A few years earlier he had carried out the Russian translation of the popular manual by Auguste Cahours, a French professor who collected the discussion surrounding the natural and artificial classifications in previous years, and offered groups of families of substances similar to those in the periodic tables of the 1860s and 1870s.
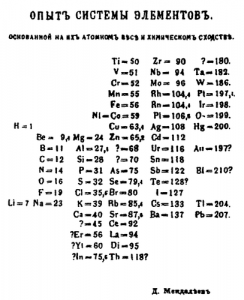
One of the first versions of Mendeleev’s periodic table (1869). Wikipedia.
Mendeleev made many versions of his periodic system. In the first versions, the elements were ordered according to groups of natural families, similar to those that appear in other manuals of the period, and in an increasing order of atomic weights according to the data available at the time. Some data was uncertain as many elements had just been discovered and there was no precise information about either their properties or their atomic weights, which caused the many question marks that appear in Mendeleev’s first tables. To compare it with the current ones it is necessary to rotate it 90 degrees because Mendeleev’s rows correspond to what is currently and usually represented in columns, that is, families of elements with similar properties. For this to be possible, Mendeleev had to leave some gaps in the table, which also appear marked with a question mark. Some of these gaps were predictions of new elements that were later confirmed by the discovery of gallium and germanium in the 1870s.
Other predictions made by Mendeleev did not have the same fate. For example, some corrections of atomic weights, necessary to maintain their periodic law, such as the weight of tellurium, were never confirmed. Nor was his prediction of an element called ‘coronium’, which should be part of the Sun’s corona and have a lower atomic mass than hydrogen, ever confirmed. In fact, Mendeleev had great difficulty in making room in his last periodic tables for the new elements found at the end of the nineteenth century, for example, noble gases. To include the new elements, many authors of the late nineteenth century reformulated the periodic table more decisively and thus made new predictions with more or less success. For example, in 1895, Julius Thomsen (1826-1909), a Danish professor, presented a model of a table that was later used by Niels Bohr in his studies on early quantum mechanics. It also served to establish a theory of the evolution of matter and the origin of the universe. This question particularly interested José Muñoz del Castillo (1850-1926), a professor from Madrid who made several new proposals for periodic tables, combining risky assumptions about the origins of the elements with notions about the evolution of the universe coming from the first studies of astrophysics. Muñoz del Castillo also left numerous gaps in his table and, at the beginning of the twentieth century, boasted about having predicted, with his table, the discovery of radium.
Contrary to the heroic narratives that present him as the prophet of the chemical order, or as a genius fond of solitaire, the previous overview shows that Mendeleev was far from being the only author who proposed periodic classifications with more or less success. Nor were his predictions exceptional. Besides, his tables do not contain all the conceptual elements of the periodic system either. In fact, many contributions were made in the years after his death, both from a theoretical point of view (concepts such as ‘atomic number’ or ‘isotope’) and experimental (especially after the discovery of radioactivity and elements after uranium). Perhaps the main novelty was the concept of atomic number, formulated in the early twentieth century by Henry Moseley (1887-1915), shortly before he died in World War I. Later on, the works of Niels Bohr and other authors related to quantum mechanics provided new ways of explaining the structure and regularities of the periodic table to promote new forms of organisation. The periodic table has been built and modified with new proposals to date, when 118 elements are already known, perhaps some more when reading these lines, twice as many as those known in Mendeleev’s time.
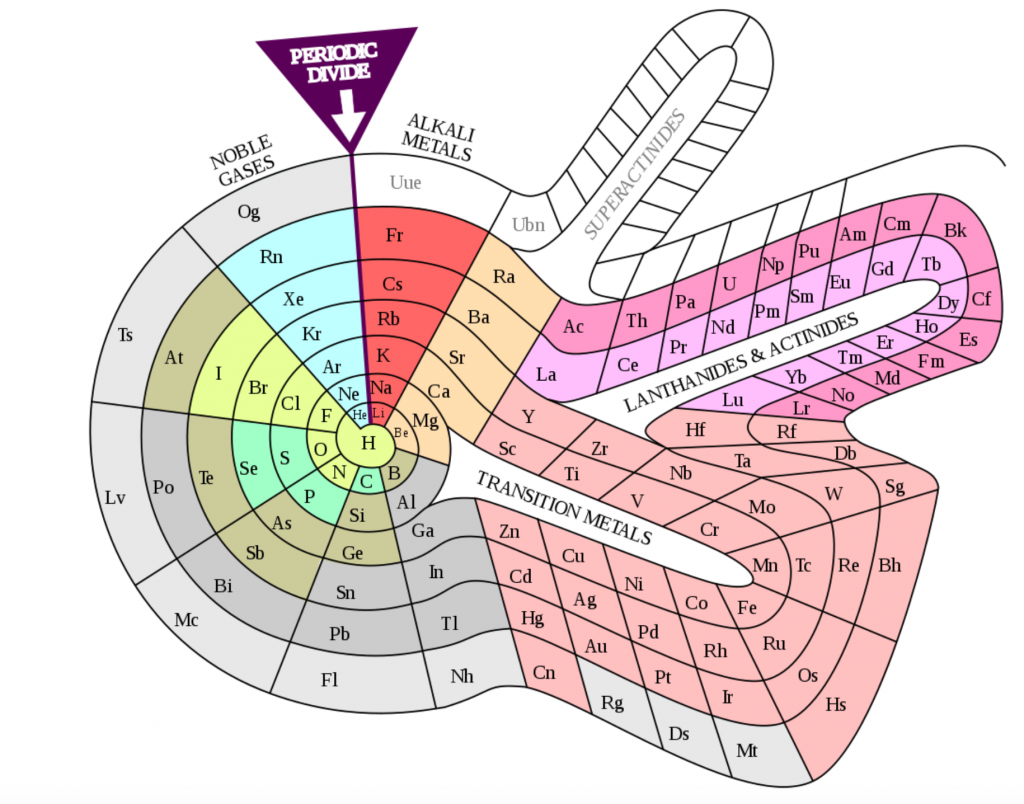
Periodic table proposed by Theodor Benfey in the 1960s, an example of the varied representations of this icon of science. Wikipedia.
Women played a prominent role in these advances, as has been pointed out in the recent publications described in the bibliography. It is well known that one of the main researchers related to the study of radioactivity and the discovery of radium was Marie Curie. There were many other women who were involved in studies related to the periodic table and the elements. Some of them discovered new elements, such as Marguerite Perey (1909-1975), who researched francium, or Ida Tacke Noddack (1896-1978), co-discoverer of rhenium together with her husband Walter Noddack and the scientist Otto Berg. The Austrian physicist Lise Meitner (1878-1968), along with the chemist Otto Hahn discovered protactinium in Berlin and made key theoretical contributions to understanding nuclear fission in the 1930s. Many other researchers, often with little recognition for their work as was the case of Meitner, made relevant contributions in the theoretical field. For example, the concept of the ‘isotope’, introduced by the British chemist Frederick Soddy (1877-1956) in 1913, was named after the proposal of the physician Margaret Todd (1859-1918) who used Greek roots to describe substances that occupied ‘the same place’ in the periodic table. For her part, Stefanie Horovitz (1877–1942), while working at the Vienna Radium Institute, provided experimental evidence of the existence of isotopes through her determination of the atomic masses of various samples of lead.
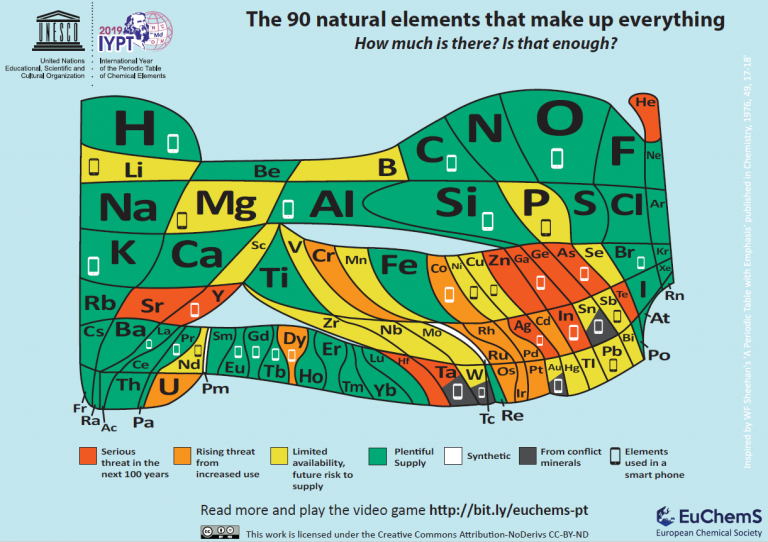
Periodic table produced by the European Chemical Society (EuChemS) to celebrate 2019. It is inspired by earlier ones and seeks to emphasize the increasing scarcity of some key elements. EuChemS.
The periodic system is, therefore, the product of a collective effort, developed over a long period of time and in various places, by a large number of people, many of whom have little presence in the most common accounts. The previous overview confirms the creative role of teaching, as has already been seen in another section. It is a collective creativity in which a large number of protagonists come together, from the teaching staff to the students, as well as the teams in charge of publishing houses and the management of educational policies. The periodic system – like its ancestor, the affinity tables – has gone beyond the academic world. It is a source of visual forms that circulate creatively across linguistic boundaries and can be managed in diverse social and cultural contexts. Since the nineteenth century, there have been numerous ways of representing it as dimensional tables and three-dimensional models. There have been controversies, some of which are still ongoing, about how it is best to visualise it, as well as about the scope and limitations of each representation. On occasions, other forms of representation of the elements have emerged (for example, regarding their greater or lesser abundance), which have been inspired by the periodic table to try to highlight problems that are not visible in traditional forms. On the other hand, beyond the limits of the scientific community, the periodic table has been transformed into an element of the popular image of chemistry.
Through secondary education, a large part of the population has become familiar with some of the standardised forms of the periodic system that can also be found in science museums and informative works. In this way, the periodic table has been a source of inspiration for literary works (such as Primo Levi’s), artistic projects and even television series (such as Breaking Bad). In short, when it is taken away from the mythology of the scientific account, the history of the periodic system offers many interesting elements to understand collective processes of scientific production and the construction of the social image of science.
José Ramón Bertomeu Sánchez
IILP-UV
How to cite this paper:
Bertomeu Sánchez, José Ramón. Classification and discipline: The order of the elements. Sabers en acció, 2021-01-05. https://sabersenaccio.iec.cat/en/classification-and-discipline-the-order-of-the-elements/.
Find out more
You can find further information with the bibliography and available resources.
Recommended reading
Bertomeu Sánchez, José Ramón. “Pedagogía Química y Circulación de la Ciencia: El Sistema Periódico de Los Elementos Durante El Siglo XIX.” En: Química: Historia, Filosofía, Educación, 45–69. Bogotá: Universidad Pedagógica; 2011.
Scerri, Eric R. La Tabla Periódica: Una Breve Introducción. Madrid: Alianza Editorial; 2013.
Studies
AA.VV. “Special Issue on Mendeleev”. Ambix. 2013; 45(2): 50-128.
Bertomeu Sánchez, José Ramón; García Belmar, A.; Bensaude-Vincent, Bernadette. “Looking for an Order of Things: Textbooks and Chemical Classifications in Nineteenth Century France.” Ambix. 2002; 49 (2): 227–51.
Bertomeu Sánchez., José Ramón «El sistema periòdic i la pedagogia química del segle XIX. La creativitat col·lectiva de les aules de ciències». Educació Química. 2019; 25: 21-31.
Brooks, Nathan M. “Dimitrii L. Mendeleev’s Principles of Chemistry and the Periodic Law of the Elements.” In Communicating Chemistry: Textbooks and Their Audiences, edited by Anders Lundgren; Bernadette Bensaude-Vincent (eds.), 295–311. Canton: Science History Pub; 2000.
Gordin, Michael D. A Well-Ordered Thing: Dmitrii Mendeleev and the Shadow of the Periodic Table. New York: Basic Books, 2004.
Gordin, Michael D. 2019. «Ordering the Elements». Science. 2019; 363 (6426): 471-73.
Kaji, Masanori. “Mendeleev’s Discovery of the Periodic Law: The Origin and the Reception.” Foundations of Chemistry. 2003; 5: 189–214.
Kaji, Masanori, Helge Kragh, and Gabor Palló, eds. Early Responses to the Periodic System. Oxford: Oxford University Press, 2015.
Kragh, Helge. “The First Subatomic Explanations of the Periodic System.” Foundations of Chemistry. 2001; 3: 129–43.
Lykknes, Annette, van Tiggelen, Brigitte, Women in Their Element: Selected Women’s Contributions to The Periodic System. New Jersey: World Scientific Publishing Co; 2019.
Lykknes, Annette, y Brigitte Van Tiggelen, eds. «The Periodic System: The (Multiple) Values of an Icon». Centaurus. 2019; 61 (4): 287-456. https://doi.org/10.1111/1600-0498.12246.
Moreno Martínez, Luis. «¿Pseudohistoria de la ciencia en los libros de texto? El caso de la tabla periódica». Publicado en SciLogs (Investigación y Ciencia). 2019.
Muñoz Bello, Rosa Muñoz; Bertomeu Sánchez, José R. “La historia de la ciencia en los libros de texto: la(s) hipótesis de Avogadro.” Enseñanza de las ciencias. 2003; 21 (1): 147–60.
Scerri, Eric R. La Tabla Periódica: Una Breve Introducción. Madrid: Alianza Editorial; 2013.
Tiggelen, Brigitte Van, y Annette Lykknes. «Celebrate the Women behind the Periodic Table». Nature. 2019; 565 (7741): 559. Versión en castellano disponible en Investigación y Ciencia.
Sources
García Belmar, Antonio.; Pellón González, Inés; Rocke, Alan. (eds.). El atomismo en química. Un Nuevo Sistema de Filosofía Química de John Dalton. Alicante: Publicaciones de la Universidad de Alicante; 2012.
Grapí, Pere; García Belmar, Antonio, eds. La Representación de Lo Invisible. Tabla de Los Diferentes Rapports Observados entre Diferentes Sustancias de Etienne-François Geoffroy. Acompañado de Un Ensayo de Ursula Klein. Alicante: Publicaciones de la Universidad de Alicante; 2012.
Halperin de Destaillats, Louis. Teoría Atómico-Molecular. Una selección de textos de Dalton, Avogadro y Ampère. Buenos Aires: Eudeba; 1965.
Mendeléiev, Dimitri. La Relació entre les propietats dels elements i llur pes atòmic. Barcelona: IEC, 2005.
Jensen, William, ed. Mendeleev on the Periodic Law: Selected Writings: 1869-1905.
Dover Books on Chemistry. Mineola: Dover; 2002.
Websites and other resources
Bertomeu Sánchez, José Ramón. “150 años del sistema periódico de Mendeléiev: la creatividad colectiva de las aulas de ciencias”. Conference given in Bogotá, July 2019. Available here.
Giunta, Carmen «Classic Papers of the History of Chemistry». [Accessed 30 April 2019]. Available here.
Leach, Mark. 2020. «The INTERNET Database of Periodic Tables». [Accessed 3 April 2020]. Available here.