—A deconstruction of ‘crucial’ experiments in the history of molecular biology.—
In the field of life sciences, the twentieth century is usually characterised as the setting for a process of molecularisation. This process has been explained in various ways. For example, the historian Pnina Abir-am has explained it as a colonisation of life sciences by exact (or ‘hard’) sciences. This colonisation would take place in a successive way and has been conditioned by the development of the great military conflicts and the leading roles played by each of these sciences in these conflicts. Chemistry would colonise life sciences from the First World War with the emergence of biochemistry. Physics would do so from the Second World War, with the emergence of molecular biology. Furthermore, in the context of the Cold War, computing and high technology would do so, thus giving rise to modern biotechnology. The molecularisation of biological sciences has also been explained based on the institutionalisation of biochemistry and genetics as disciplines in the early twentieth century, and their subsequent encounter in the emergence of molecular biology. Molecular biology could perhaps be understood as a discipline in its own right initially or from the 1960s. However, finally, and especially from the 1980s, it acquired the identity of an ultra-discipline, from which it would influence the entire field of life sciences.
For biochemistry and genetics to be consolidated as disciplines, among many other things, both had to be identified with their own intellectual space defined by specific vocabulary, distinctive methodology and some questions or problems that distinguished them from other areas. While biochemistry emphasised protein, genetics focused on the gene and, in this way, the possible common area remained a border area with little or no relevance to the respective disciplines. Thus, for example, essential authors for the development of genetics such as Thomas Morgan (1866-1945) consciously refused to ask about the mechanisms of gene action or the chemical nature of such genes. For historians like Michel Morange, molecular biology emerged precisely when the possibility of working in this no man’s land, which was halfway between biochemistry and genetics, opened up. One of the key studies for building bridges between these disciplines – and, from this perspective, fundamental for the birth of molecular biology – was that developed by George Beadle (1903-1989) and Edward L. Tatum (1909-1975) in the early 1940s. With their experiment, Beadle and Tatum would establish that genes control enzymatic activities and that there was a different gene for each enzyme. These conclusions were highly valued and they were finally awarded the Nobel Prize for them.
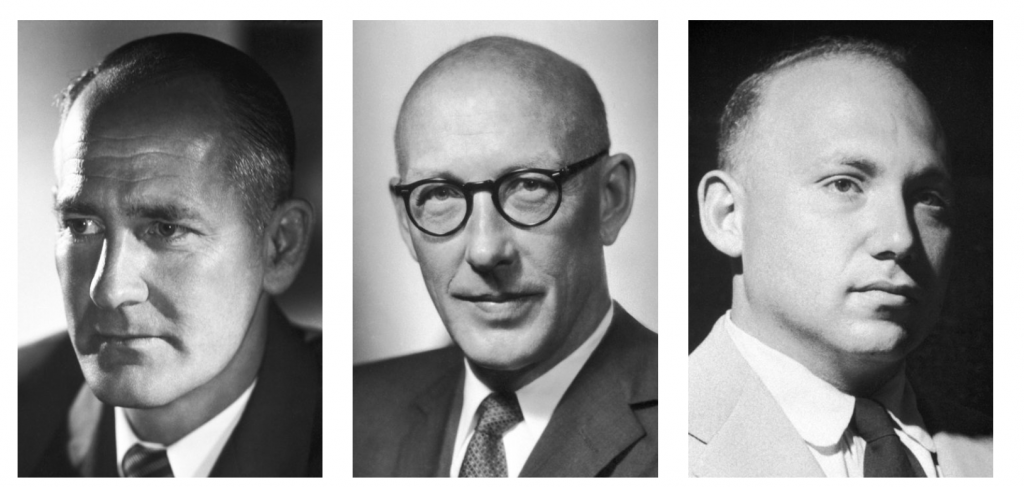
George Wells Beadle (left) and Edward Lawrie Tatum (centre) won the Nobel Prize in Physiology and Medicine in 1958 for their discovery of “the role of genes in the regulation of chemical phenomena”. They shared the prize with Joshua Lederberg (right), who was awarded the prize for his studies on genetic recombination and the organisation of genetic material in bacteria. The Nobel Prize.
The relationship between genes and enzymes studied by George Beadle had been previously been sensed by the scientific community, but it had never been studied successfully, partly because it was an issue that remained in this previously described no man’s land. Settled in his laboratories at Stanford University, Beadle hired a specialist in microbiology (Edward Tatum) and together they developed the experiment considered to be crucial, which allowed him to establish his famous aphorism ‘one gene, one enzyme’. They decided to use a new model organism, Neurospora crassa, a fungus that was especially interesting for their study because many generations could be obtained in a short time and, in addition, it had a haploid phase (in which the organism contains only one copy of each gene), with which all mutations could be observed, regardless of whether the mutant allele was recessive or not. It also went through a diploid phase that allowed the sexual reproduction necessary in the studies of classical or Mendelian genetics. In addition, it was an organism that, under normal conditions, could survive well in a minimal culture medium because it could biosynthesise all the elements it required to survive from the biotin, salts and sugars present in that minimal medium.
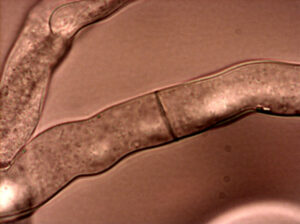
Neurospora crassahyphae. Wikimedia.
In the course of their experimentation, Beadle and Tatum irradiated strains of Neurospora crassa with X-rays to induce mutations. They thus observed that some of the new strains could no longer survive in the minimal medium, but had a new requirement. For example, some of the mutant strains were not able to survive in a medium that did not contain the amino acid arginine. In these cases, they assumed that the mutation had affected one of the enzymatic activities involved in the arginine biosynthesis pathway. By working with these strains, they determined the enzymatic activity that had been affected for each mutant and associated it with the alteration of a gene. They concluded that there was a gene for every enzymatic activity. In any case, beyond the particularities of the experiment, there was a series of interesting questions around it, which allow us to better understand its development as a ‘crucial experiment’ and its inclusion in the basic or applied science of its time.
More traditional historiography and poor historical dissemination tend to emphasise crucial experiments as a source of revolutions or turning points in the history of science. These experiments would bring to light new indisputable facts that would clearly establish the superiority of modern interpretations. However, the history and sociology of twentieth-century science, with their notion of empirical underdetermination of scientific theories, have cast serious doubt on the existence of such crucial experiments. In the case studied, influential authors such as Max Delbrück stated that the Beadle and Tatum’s results were compatible with both the ‘one gene, one enzyme’ relationship, and with the possibility that each enzyme was produced by different genes (as in fact happens on many occasions). In the end, everything indicates that the experiment was not so important for the conclusion formulated in the famous aphorism, but because it broke disciplinary barriers and turned genetics into an interesting field for the biochemical study of metabolic pathways. On the other hand, although the research is usually presented as typical of pure science, its development was only possible in the context of a World War because its authors skilfully presented it as a work of applied science, as historians such as Lily Kay have shown. Beadle and Tatum claimed to be able to construct a set of mutant microorganisms through their experiment, which could be used to identify the presence of specific nutrients in food or drugs.
The case studied shows, therefore, how the boundaries between applied and pure science are more blurred when viewed from a historical perspective. The definition of studies as applied or pure science is not a minor issue. Authors such as Dominique Pestre have emphasised that if nineteenth-century science has been presented as pure on so many occasions, it has been in an interested way, usually in a search for authority, veracity or disinterestedness that was not considered part of the virtues of applied science. On the other hand, historians such as Michel Morange have proposed a periodisation of the history of molecular biology that seems to be inspired by the much-discussed linear model of technological innovation. Morange was referring to an initial stage that would comprise approximately the 1940s, 1950s and 1960s, in which the conceptual bases of molecular biology were established (which was to be understood as a stage of pure science) and from the beginning of the 1970s a stage for the development of operational techniques (a stage of applied science) would begin. However, the analysis of that experiment in this section, based on the works of Lily Kay, clearly contradicts this periodisation and its apparent connection with the linear model. Beadle and Tatum’s experiment thus raises a number of questions for rethinking the historical development of molecular biology.
Ximo Guillem Llobat
IILP-UV
How to cite this paper:
Guillem Llobat, Ximo. One gene, one enzyme. Sabers en acció, 2021-01-29. https://sabersenaccio.iec.cat/en/one-gene-one-enzyme/.
Find out more
You can find further information with the bibliography and available resources.
Recommended reading
Judson, Horace Freeland. The eighth day of creation. Makers of the Revolution in Biology. Plainview, NY: Cold Spring Harbor Laboratory Press; 1996 (first published in 1979).
Morange, Michel. Histoire de la biologie moléculaire. Paris: Découverte; 1994
Olby, Robert. The path to the double helix. The discovery of DNA. Seattle: University of Washington Press; 1974.
Studies
Abir-am, Pnina. The Molecular Transformation of Twentieth-Century Biology. In: Krige, John; Pestre, Dominique, eds. Science in the twentieth century. Amsterdam: Harwood Academic Publishers; 1997, p. 495-524.
Bowler, Peter. The mendelian revolution: the emergence of hereditian concepts in modern science and society. Baltimore: Johns Hopkins University; 1989.
Creager, Angela N. H. The life of a virus: Tobacco Mosaic Virus as an experimental model, 1930-1965. Chicago: Chicago University Press; 2002.
De Chadarevian, Soraya. Of worms and programmes: Caenorhabditis elegans and the study of development. Studies in History and Philosophy of Science Part C: Studies in History and Philosophy of Biological and Biomedical Sciences. 1998; 29: 81-105.
Holmes, Frederic Lawrence. Meselson, Stahl, and the replication of DNA: A history of the most beautiful experiment in biology. New Haven: Yale University Press, 2002.
Judson, Horace Freeland. The eighth day of creation. Makers of the Revolution in Biology. Plainview, NY: Cold Spring Harbor Laboratory Press; 1996 (first published in 1979).
Kamminga, Harmke. Biochemistry, Molecules and Macromolecules. In: Krige, John; Pestre, Dominique, eds. Science in the twentieth century. Amsterdam: Harwood Academic Publishers; 1997, p. 525-546.
Kay, Lily E. Selling Pure Science in Wartime: The Biochemical Genetics of G.W. Beadle. Journal of the History of Biology. 1989; 22: 73-101.
Kohler, Robert. From medical chemistry to biochemistry: The making of a biomedical discipline. Cambridge: Cambridge University Press; 1982.
Kohler, Robert. Lords of the fly: drosophila genetics and the experimental life. Chicago: University of Chicago Press; 1994.
Morange, Michel. Histoire de la biologie moleculaire. Paris: Découverte; 1994.
Nye, Mary Jo. From chemical philosophy to theoretical chemistry: dynamics of matter and dynamics of disciplines, 1800-1950. Berkeley: University of California Press; 1994.
Pestre, Dominique. Ciència, diners i política: assaig d’interpretació. Santa Coloma de Queralt: Obrador Edèndum, Publicacions URV; 2008.
Olby, Robert. The path to the double helix. The discovery of DNA. Seattle: University of Washington Press; 1974.
Rheinberger, Hans-Jörg. Recent science and its exploration: the case of molecular biology. Studies in History and Philosophy of Biological and Biomedical Sciences. 2009; 40: 6-12.